
Solving and refining the crystal structures of solids synthesized directly from elements in laser-heated conventional DACs 10– 15 at pressures as high as up to about two megabars 12, 16 became possible owing to the synergy of our expertise both in generating pressures of several megabars 3, 17, 18 (for details see Supplementary Information section ‘Brief overview of the double-stage DAC (dsDAC) technique’) and in single-crystal X-ray diffraction (XRD) at ultrahigh pressures, which were pioneered a few years ago 19, 20. Very recent examples are the discovery of a new nitrogen allotrope 9, bp-N, which resolved a puzzle in our understanding of the high-pressure behaviour of pnictogen family elements, and the synthesis of a plethora of novel transition metal nitrides and polynitrides 10– 15, including metal–inorganic frameworks 11, 15, which are a new class of compounds featuring open porous structures at megabar compression. Recent developments in the diamond anvil cell technique, and, particularly, the invention of double-stage and toroidal diamond anvil cells (dsDACs and tDACs) 3, 7, 8, have enabled breakthroughs in the synthesis of materials and the study of structure–property relationships at high and ultrahigh pressures. Compression is known to facilitate metal-to-insulator transitions 4, superconductivity 5 and new ‘super’ states of matter 6. This gives rise to various phenomena relevant for a broad range of scientific disciplines and technological applications, from fundamental understanding of the Universe to targeted design of advanced materials. The state of matter is strongly affected by variations in chemical composition and external parameters such as pressure and temperature, enabling tuning of material properties. Full chemical and structural characterization of the materials, realized using synchrotron single-crystal X-ray diffraction on microcrystals in situ, demonstrates the capabilities of the methodology to extend high-pressure crystallography to the terapascal regime. We apply this method to realize pressures of about 600 and 900 gigapascals in a laser-heated double-stage diamond anvil cell 3, producing a rhenium–nitrogen alloy and achieving the synthesis of rhenium nitride Re 7N 3-which, as our theoretical analysis shows, is only stable under extreme compression. Here we report on a methodology developed to enable experiments at static compression in the terapascal regime with laser heating.
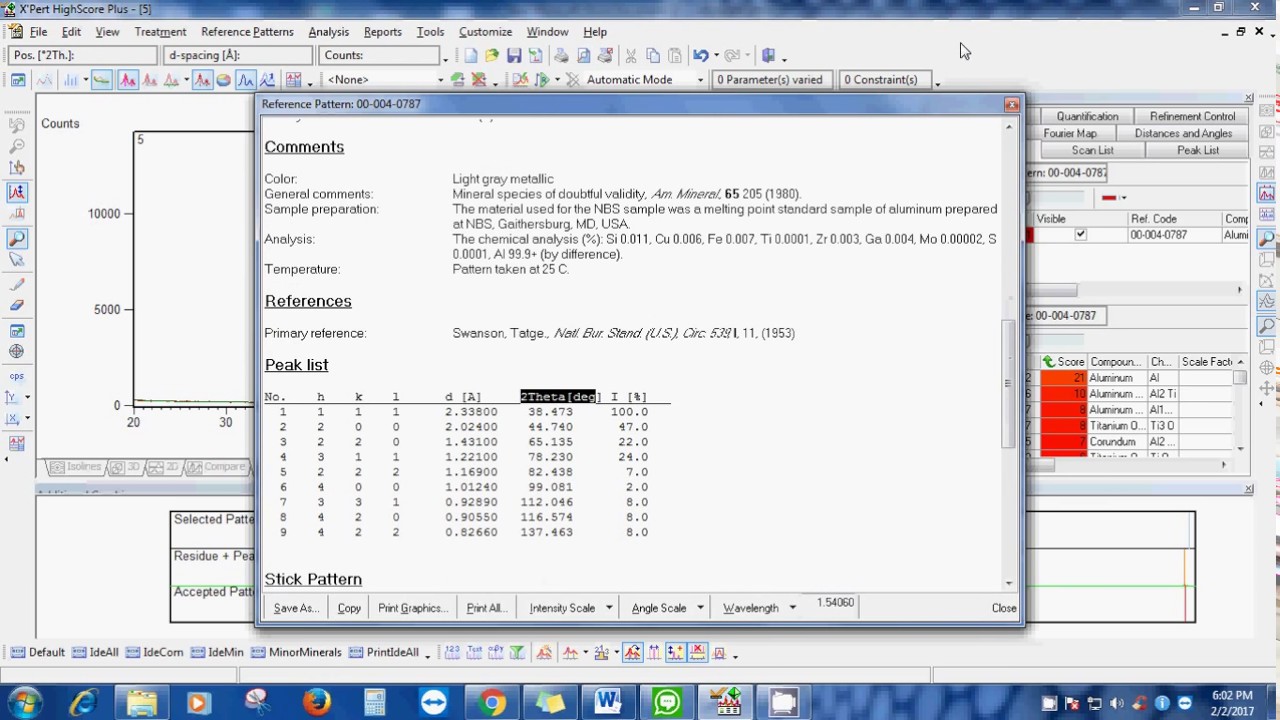
Hitherto, their synthesis and investigation above 200 gigapascals have been hindered both by the technical complexity of ultrahigh-pressure experiments and by the absence of relevant in situ methods of materials analysis. Theoretical modelling predicts very unusual structures and properties of materials at extreme pressure and temperature conditions 1, 2.
